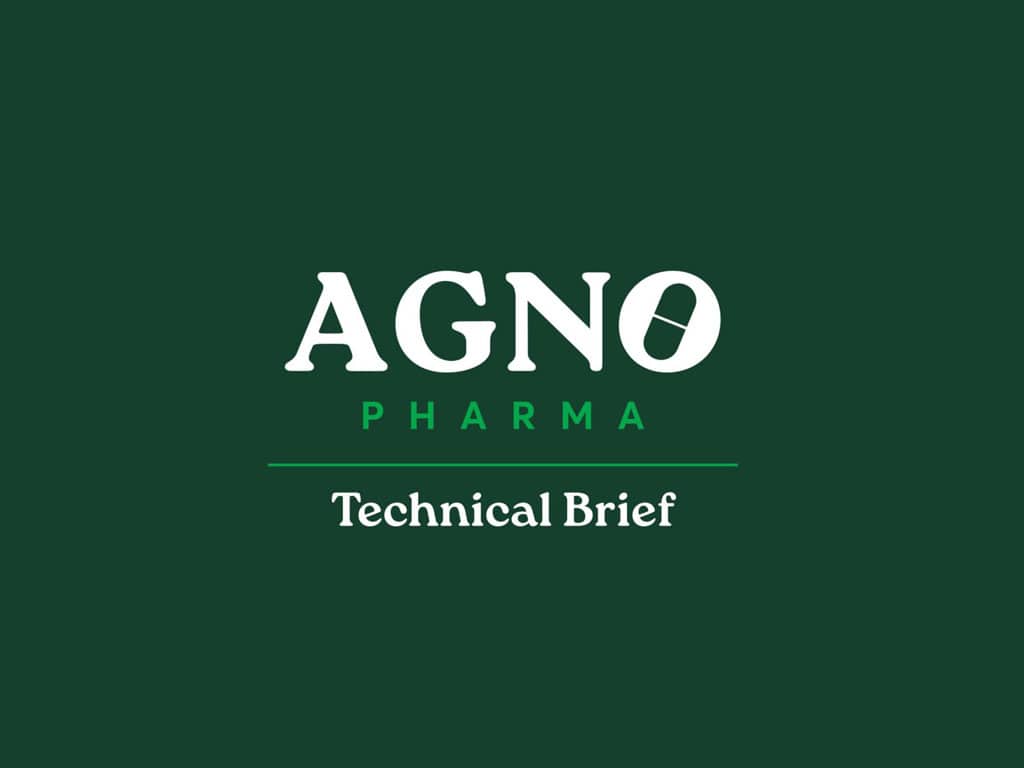
Emulsions and Emulsifications
Emulsions are used in a wide variety of industrial and pharmaceutical products including ocular, topical, mucosal, intravenous, intramuscular, and oral products. Emulsions are also used as precursors to prepare polymer microparticles, solid lipid nanoparticles, inorganic nanoparticles and oilfilled microcapsules and have been developed as precursors to magnetic particles for imaging, diagnostics and drug delivery. Even with their widespread use, they remain an underutilized drug product format.
In a drug product the emulsion may be:
- The active component itself
- An activity-enhancer for a co administered active pharmaceutical ingredient (API), either acting passively as a solvent/stabilizing system or actively as a penetration/absorption enhancing system
- Pharmaceutically inactive and simply provide an elegant vehicle for an API formulation
In its simplest form, an emulsion is a mixture of two immiscible liquids (usually an organic “oil” and water) in which one liquid (the dispersed phase) is in the form of microscopic droplets dispersed in the other (continuous) phase. Emulsions also contain emulsifiers – materials that concentrate at the phase interface to lower the interfacial tension. Emulsifiers reduce the energy required to break the dispersed phase into droplets and prevent them from coalescing by generating a repulsive force or a physical barrier between them.
Emulsions that are formed by dispersing a water-immiscible liquid into an aqueous phase are termed oil-inwater (o/w), while those having aqueous droplets dispersed in a continuous oil phase are termed water-in-oil (w/o). More complex “triple” emulsions have dispersed droplets that contain smaller droplets of continuous phase material. A triple emulsion with water dispersed in oil droplets, with themselves then dispersed in water, is referred to as a water-in-oil-in-water emulsion (w/o/w) and the less common reverse analogue is termed oil-in-water-inoil (o/w/o). These emulsion types are shown schematically in Figure 1.
Most emulsions contain droplets with a mean diameter of >1µm, however mini-emulsions and nano-emulsions can be formed with droplet sizes in the 100-500 nm range, and with proper formulation, highly stable microemulsions can be prepared having droplets as small as a few nanometers. A Pickering emulsion is one stabilized with ultrafine solid particles rather than molecular emulsifiers. The stabilizing particles are selected or engineered to have a contact angle with water of close to 90° so that they are wetted by the continuous and dispersed phases. Therefore, they remain at the droplet interface where they can provide a barrier to droplet coalescence.
Emulsification
The Gibb’s free energy change, ΔG, on forming an emulsion from two bulk liquids is given by the familiar equation: ΔG=ΔH–TΔS, where ΔH is enthalpy change, T is temperature, and ΔS is entropy change.
100 ml of an o/w emulsion containing 10% w/w of oil in the form of 1 µm droplets, contains 2 x 1013 droplets, having a combined surface area of 60 m2
Without going deeply into the relative energetic contributions, the free energy of emulsification is positive. The first corollary of this is that emulsification is rarely a spontaneous process, and hence requires the input of energy. This energy usually comes from mechanical shear provided by various types of mixers, and the final droplet size of the emulsion is dependent on not only the chemistry but also the amount of energy applied. Simple paddle-type mixers are suitable when the required droplet size is above ~10 µm but to make smaller droplets, higher shear is generally required. High shear rotor-stator mixers are commonly used. These operate by pumping the emulsion phases through the narrow gap between a perforated cylinder (the stator) and the blades of a rapidly rotating stirrer housed inside it (the rotor) where the forces are sufficient to make droplets as small as around 1 µm (Figure 2).
To make even smaller nano-sized droplets, at small scale, ultrasonic devices can be used, but they are generally not practical to scale up. High-pressure homogenizers are readily scaled and can also produce emulsions with nano-sized droplets. The emulsion phases are pumped together under high pressure into a small volume or through a small orifice – the interaction chamber where pressures in the tens of thousands of PSI create very high shear forces (Figure 3).
Emulsions with an average droplet size as small as 0.2 µm can be formed this way, which is significant as these are amenable to sterilization by filtration through a 0.2 µm filter.
Emulsions are prepared by adding one phase to the other over time, with one or both phases containing emulsifier, and applying shear. In some cases, one or both phases are heated prior to mixing to aid emulsification. The phases are mixed until the mean droplet size is within specification. With overhead stirrers this is simply a matter of time, whereas with a high-pressure homogenizer each portion of the mixture sees only brief mixing as it passes through the interaction chamber, so once collected, the product of the first emulsification pass-through may then need to be re-fed through the machine until the desired size is obtained. It is not uncommon to require multiple passes to achieve the desired droplet size.
Stabilization
A second corollary of the positive free energy change of emulsification is that most emulsions are thermodynamically unstable, and over time the droplets tend to coalesce to reform the separate bulk oil and aqueous phases. To prepare emulsions with the shelf life stability required for a pharmaceutical product (1-3+ years), the correct choice of emulsifiers must be made and/or polymeric stabilizers must be added afterwards.
The most common emulsifiers and emulsion stabilizers are amphiphilic molecules with a polar group, the hydrophile, attached to a non-polar group, the hydrophobe, (Figure 4).
They may be anionic (e.g. alkyl sulfates, sulfonates and phosphates, fatty acids), cationic (e.g. quaternary alkyl ammonium salts), or non-ionic (e.g. polysorbates). These are relatively small molecules that concentrate at the oiI-water interface to prevent droplets from coalescing. The anionic and cationic emulsifiers prevent droplet coalescence by imparting static charge to the droplets which renders them mutually repulsive and prevents close approach. The nonionic emulsifiers create a physical barrier to droplet coalescence. Higher molecular weight polymers dissolved in the continuous phase also help to stabilize the droplets against coalescence in a similar way to that of the non-ionic emulsifiers.
Microemulsions are a special case that contain such high levels of emulsifiers, and often co-emulsifiers (usually >10 wt% of the formulation), that the interfacial tension between the dispersed and continuous phases is near-zero. This means TΔS > ΔH and ΔG < 0 therefore they form spontaneously and are thermodynamically stable.
Sometimes, if the dispersed phase has finite solubility, the continuous phase material diffuses from the smaller droplets to the larger ones. These larger droplets grow at the smaller droplets’ expense and tend to increase the average droplet size over time. This process, called Ostwald ripening, can be prevented by dissolving a solute into the dispersed phase that is completely insoluble in the continuous phase, before emulsification. This is effective because any loss of the dispersed phase liquid from the small droplets by Ostwald ripening causes an increase in the chemical potential of the solute in those droplets, therefore the dispersed phase material diffuses back to the small droplets.
Gravitational droplet sedimentation or creaming occurs when the densities of the two phases of the emulsion are dissimilar, and the viscosity of the emulsion is low enough to allow the droplets to move. To prevent phase separation in cases where the phase densities cannot be changed, it is common to increase the viscosity of the emulsion – either by dissolving thickeners in the continuous phase or formulating the emulsion with a high volume fraction of dispersed phase so that droplet packing itself gives rise to higher viscosity. Close packing of monodisperse non-deformable spheres in three dimensions gives a theoretic maximum of 74% volume occupancy of the dispersed phase. In specially formulated high internal phase emulsions (HIPEs), the dispersed phase comprises more than 74% of the formulation, permissible because of the broad droplet size distribution, stable interfaces, and the deformable nature of the liquid droplets. HIPEs have significant viscosity and do not tend to separate under gravity.
The stability of o/w emulsions that are stabilized by charged emulsifiers is dependent on the electrolyte concentration. As with most other colloidal systems, emulsions are destabilized by high concentrations of electrolytes, especially when they contain multivalent ions, and droplet aggregation and coalescence are observed. This is well described by DLVO theory. The use of non-ionic emulsifiers and polymeric stabilizers with, or instead of, charged emulsifiers greatly increases emulsion stability in the presence of salts. This is due to that fact that destabilization is a result of the salt-induced reduction in electrostatic repulsion between charged droplets made with ionic emulsifiers.
Addition of API
APls can be added to the emulsion is several ways. If the emulsion is simply to be used as an elegant vehicle for API delivery, for example topically, and the API is soluble in one of the liquid phases, then it can be added to that phase before emulsification. If it is to be added to the continuous phase it may be added after a placebo emulsion has been made. If the API is to be added to the continuous phase, but is not soluble in it, it can be simply dispersed or added as a solution in a solvent that is miscible with the continuous phase. Some APls are surface active and are added initially, as they can aid in the emulsification of the dispersed phase. When proteins are used, for example an antigen or antibody, the protein is best added last, as the high shear forces can otherwise denature it. Care must also be taken as adsorption of proteins to hydrophobic surfaces such as dispersed oil droplets, can cause them to undergo conformational change and denature, or present otherwise hidden off-target epitopes to the immune system cells.
To prepare a consistent quality product, emulsion-based pharmaceutical formulations are characterized by a number of techniques typically used for the characterization of colloids. These include particle size distribution, (which can be a complex measurement if the API is in particulate form, with a similar particle size to that of the emulsion dispersed phase droplets), electrical charge of the droplets (via zeta potential measurements), creaming or sedimentation rate (via Turbiscan), phase separation (by eye), and rheology. For development-stage studies, a parallel plate rheometer is used to determine viscosity and complex flow characteristics, whereas in production, the Brookfield viscometer is a simpler QC tool.
Conclusions
Emulsions offer the formulator an elegant passive or active drug delivery vehicle. Knowledge of the potential mechanisms for formulation instability and proper formulation can yield formulations with suitable shelf life. By selecting suitable excipients, regulatory approval can be straightforward, leading to new products that have improved efficacy, safety, compliance, and can offer patent extension for life cycle management.